In the future we won’t edit genomes—we’ll just print out new ones
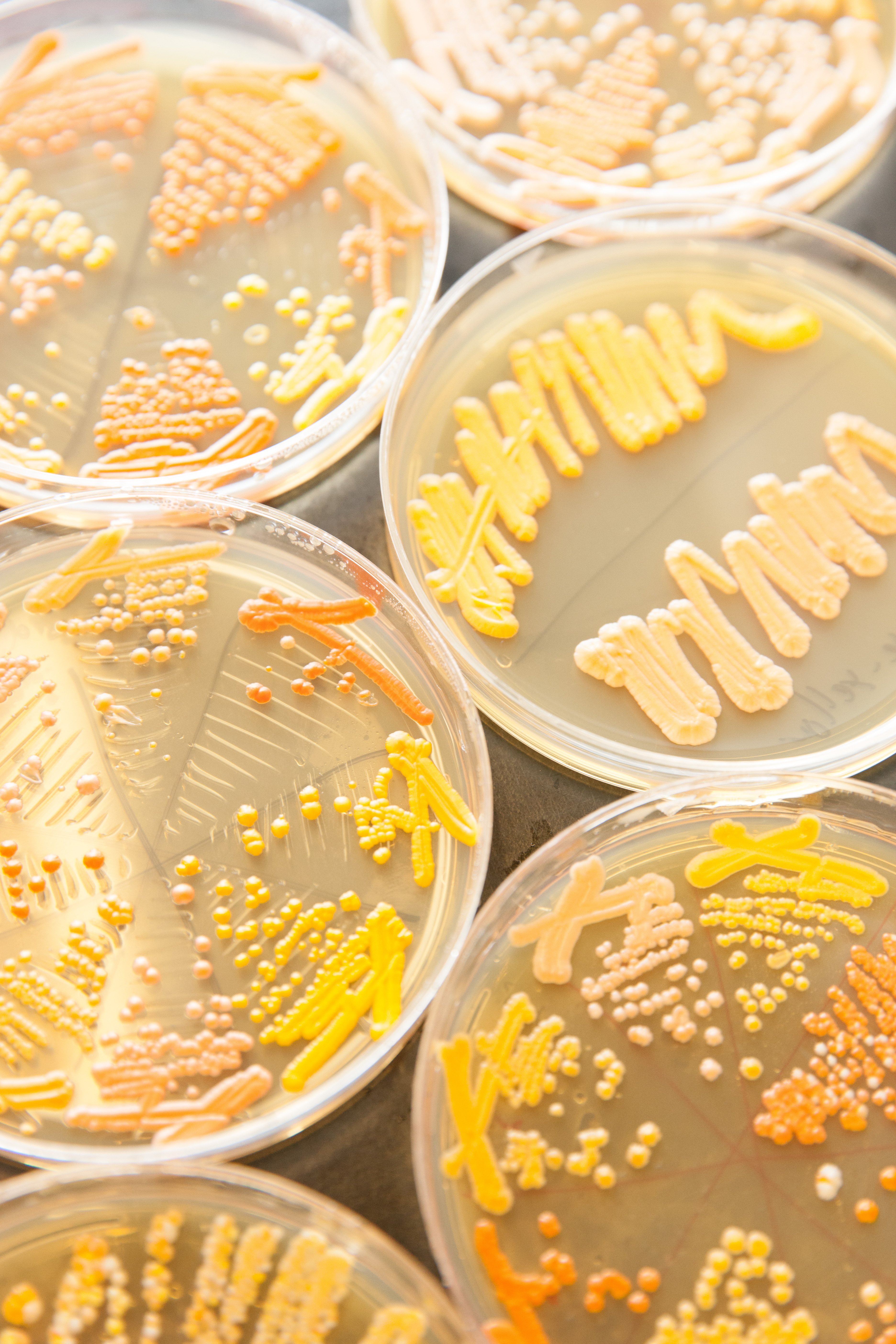
At least since thirsty Sumerians began brewing beer thousands of years ago, Homo sapiens has had a tight relationship with Saccharomyces cerevisiae, the unicellular fungus better known as brewer’s yeast. Through fermentation, humans were able to harness a microscopic species for our own ends. These days yeast cells produce ethanol and insulin and are the workhorse of science labs.
That doesn’t mean S. cerevisiae can’t be further improved—at least not if Jef Boeke has his way. The director of the Institute for Systems Genetics at New York University’s Langone Health, Boeke is leading an international team of hundreds dedicated to synthesizing the 12.5 million genetic letters that make up a yeast’s cells genome.
In practice, that means gradually replacing each yeast chromosome—there are 16 of them—with DNA fabricated on stove-size chemical synthesizers. As they go, Boeke and collaborators at nearly a dozen institutions are streamlining the yeast genome and putting in back doors to let researchers shuffle its genes at will. In the end, the synthetic yeast—called Sc2.0—will be fully customizable.
“Over the next 10 years synthetic biology is going to be producing all kinds of compounds and materials with microorganisms,” says Boeke. “We hope that our yeast is going to play a big role in that.”
Think of the project as something like Henry Ford’s first automobile—hand built and, for now, one of a kind. One day, though, we may routinely design genomes on computer screens. Instead of engineering or even editing the DNA of an organism, it could become easier to just print out a fresh copy. Imagine designer algae that make fuel; disease-proof organs; even extinct species resurrected.

“I think this could be bigger than the space revolution or the computer revolution,” says George Church, a genome scientist at Harvard Medical School.
Researchers have previously synthesized the genetic instructions that operate viruses and bacteria. But yeast cells are eukaryotic—meaning they confine their genomes in a nucleus and bundle them in chromosomes, just as humans do. Their genomes are also much bigger.
That’s a problem because synthesizing DNA is still nowhere near as cheap as reading it. A human genome can now be sequenced for $1,000, with the cost still falling. By comparison, to replace every DNA letter in yeast, Boeke will have to buy $1.25 million worth of it. Add labor and computer power, and the total cost of the project, already under way for a decade, is considerably more.
Along with Church, among others, Boeke is a leader of GP-write, an organization advocating for international research to reduce the cost of designing, engineering, and testing genomes by a factor of a thousand over the next decade. “We have all kinds of challenges facing ourselves as a species on this planet, and biology could have a huge impact on them,” he says. “But only if we can drive down costs.”
Bottom up
A scientist named Ronald Davis at Stanford first suggested the possibility of synthesizing the yeast genome at a conference in 2004—though initially, Boeke didn’t see the point. “Why would anyone want to do this?” he recalls thinking.
But Boeke came around to the idea that manufacturing a yeast genome might be the best way to comprehend the organism. By replacing each part, you might learn which genes are necessary and which the organism can live without. Some team members call the idea “build to understand.”
“It’s a different take on trying to understand how living things work,” says Leslie Mitchell, a postdoctoral fellow in the NYU lab and one of the main designers of the synthetic yeast. “We learn what gaps in our knowledge exist in a bottom-up genetic approach.”
Joel Bader, a computer scientist at Johns Hopkins, signed on to develop software that let scientists see the yeast chromosomes on a screen and keep track of versions as they changed, like a Google Docs for biology. And in 2008, to make the DNA, Boeke launched an undergraduate course at Hopkins called “Build a Genome.” Students would learn basic molecular biology as each one assembled a continuous stretch of 10,000 DNA letters that would go toward the synthetic-yeast project. Later, several institutions in China joined to share the workload, along with collaborators in Britain, Australia, and Japan.
“We assign chromosomes to individual teams, like assigning a chapter of a book, and they have the freedom to decide how to do it, as long as it’s based 100 percent on what we design,” says Patrick Cai, a synthetic biologist at the University of Manchester and the yeast project’s international coordinator.
Next steps
It took Boeke and his team eight years before they were able to publish their first fully artificial yeast chromosome. The project has since accelerated. Last March, the next five synthetic yeast chromosomes were described in a suite of papers in Science, and Boeke says that all 16 chromosomes are now at least 80 percent done. These efforts represent the largest amount of genetic material ever synthesized and then joined together.
It helps that the yeast genome has proved remarkably resilient to the team’s visions and revisions. “Probably the biggest headline here is that you can torture the genome in a multitude of different ways, and the yeast just laughs,” says Boeke.

Boeke and his colleagues aren’t simply replacing the natural yeast genome with a synthetic one (“Just making a copy of it would be a stunt,” says Church). Throughout the organism’s DNA they have also placed molecular openings, like the invisible breaks in a magician’s steel rings. These let them reshuffle the yeast chromosomes “like a deck of cards,” as Cai puts it. The system is known as SCRaMbLE, for “synthetic chromosome recombination and modification by LoxP-mediated evolution.”
The result is high-speed, human-driven evolution: millions of new yeast strains with different properties can be tested in the lab for fitness and function in applications like, eventually, medicine and industry. Mitchell predicts that in time, Sc2.0 will displace all the ordinary yeast in scientific labs.
The ultimate legacy of Boeke’s project could be decided by what genome gets synthesized next. The GP-write group originally imagined that making a synthetic human genome would have the appeal of a “grand challenge.” Some bioethicists disagreed and sharply criticized the plan. Boeke emphasizes that the group will “not do a project aimed at making a human with a synthetic genome.” That means no designer people.
Ethical considerations aside, synthesizing a full human genome—which is over 250 times larger than the yeast genome—is impractical with current methods. The effort to advance the technology also lacks funding. Boeke’s yeast work has been funded by the National Science Foundation and by academic institutions, including partners in China, but the larger GP-write initiative has not attracted major support, other than a $250,000 initial donation from the computer design company Autodesk. Compare that with the Human Genome Project, which enjoyed more than $3 billion in US funding.
“This is a revolution we don’t want to fall behind on,” says Church. “If the federal government and all 50 states don’t want to do this, we will reap what we sow. We will be left behind.”
In the meantime, the labor goes on, base pair by base pair. Amid the journal covers and team photographs, Boeke has a quote on the door of his office, attributed to the geneticist Theodosius Dobzhansky: “Nothing in biology makes sense except in the light of evolution.” Whatever grand project succeeds Sc2.0—perhaps synthesizing the genome of a mouse, or engineering pigs to develop safe organs for human transplant—it will increasingly be humans directing that evolution. If that’s the case, Sc2.0 may end up being the second most important achievement ever to feature yeast—after beer.
Deep Dive
Biotechnology and health
How scientists traced a mysterious covid case back to six toilets
When wastewater surveillance turns into a hunt for a single infected individual, the ethics get tricky.
An AI-driven “factory of drugs” claims to have hit a big milestone
Insilico is part of a wave of companies betting on AI as the "next amazing revolution" in biology
The quest to legitimize longevity medicine
Longevity clinics offer a mix of services that largely cater to the wealthy. Now there’s a push to establish their work as a credible medical field.
There is a new most expensive drug in the world. Price tag: $4.25 million
But will the latest gene therapy suffer the curse of the costliest drug?
Stay connected
Get the latest updates from
MIT Technology Review
Discover special offers, top stories, upcoming events, and more.