Hacking HIV
On a May afternoon in 2008, Bruce Walker and Terry Ragon ’71 paid a recruiting visit to MIT. Walker is a Harvard Medical School physician who has studied HIV for three decades; Ragon, the founder and CEO of a software company called InterSystems, was about to bankroll a new $100 million research institute to develop HIV vaccines, with Walker at its head.
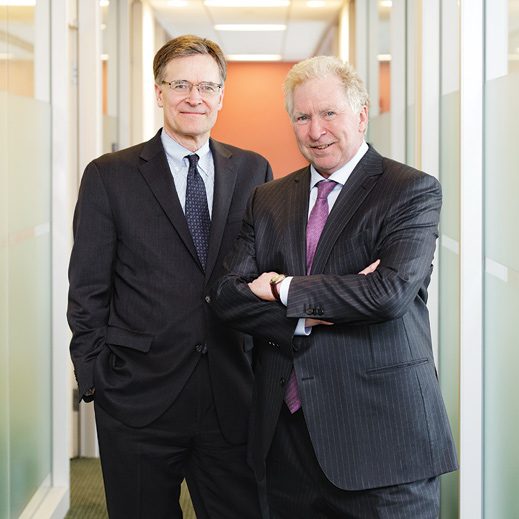
About 20 MIT faculty members came to hear Walker and Ragon’s pitch for help with their project. One of those was Arup Chakraborty, a professor of chemical engineering who was intrigued by the mission of what would be known as the Ragon Institute. He had worked in immunology for almost a decade, but he had never delved into HIV research, which had been an insular field. “Moreover, I didn’t know what I could possibly contribute,” Chakraborty recalls.
After several brainstorming sessions, Walker thought Chakraborty might be able to get to the bottom of something that had been vexing HIV researchers: people whose immune systems are naturally able to fight off HIV infection are also prone to autoimmune disorders. The phenomenon seemed unlikely to be mere coincidence. Perhaps Chakraborty, who uses computational models to study how cells in the immune system distinguish between foreign invaders and the body’s own cells, could figure out the connection.
About a year later, Chakraborty published his first paper on HIV, which focused on immune agents known as T cells. In certain people, he found, the body’s process for weeding out T cells that might attack healthy cells isn’t completely effective. But the T cells that evade this process are especially effective at fighting HIV.
“To me, that’s a great example of something that never would have happened without the community that was developed with the Ragon Institute,” says Walker.
Giving scientists, engineers, and physicians free rein to tackle HIV is the mission of the Phillip T. and Susan M. Ragon Institute of Massachusetts General Hospital, MIT, and Harvard. Since it opened, in 2009, researchers from the three institutions have worked together—and with other researchers around the world—to attack the problem from every angle.
From the outset, Walker wanted to partner with researchers outside the usual realm of virology and immunology because he didn’t think the traditional funding channels were supporting enough novel research. “I would frequently get together with people and talk about a potential collaboration with somebody outside the HIV field, and it would seem like a great idea, but it never went anywhere because we were never able to fund it,” he says. “We wanted to get a bunch of people together and really license them to work on this problem and measure success not in terms of papers published, but in terms of assault on the goal.”
This approach will have implications far beyond HIV research, says Chakraborty, now the director of MIT’s Institute for Medical Engineering and Sciences (IMES), an interdisciplinary center launched last summer (see sidebar, below). “There’s a lot of talk around the world, especially in this country, about getting this kind of work at the interface of disciplines supported,” he says. “But personally I don’t know of another example where basic scientists and clinicians have worked in such synergy as the Ragon Institute.”
Identifying the virus’s weaknesses
Antiretroviral drugs have been so effective in treating AIDS that “there’s a bit of complacency about it” in the United States, Walker says. “The problem is, the drugs have to be taken very reliably in order for resistance not to develop. Another, bigger problem is that in the rest of the world there are limited resources and limited drugs available.” Fewer than 25 percent of HIV-infected people worldwide had access to the drugs in 2011, according to the Joint United Nations Programme on HIV/AIDS.
Given those facts, Walker believes, the only way to eradicate HIV is to develop an effective vaccine. But HIV is an elusive target: it mutates much more rapidly than most viruses—even influenza, for which vaccines must be redesigned every year.
Vaccines that provoke an immune response against any of HIV’s proteins rapidly become useless as the proteins evolve. However, a few of the amino acids that make up HIV proteins remain unchanged in almost all strains, suggesting that they are essential to the virus’s survival. In recent years, vaccine designers have tried to target these amino acids. But they’ve had limited success, because amino acid substitutions elsewhere in the protein can help overcome the vaccine’s effects.
So Chakraborty looked for groups of amino acids in HIV proteins that evolve together, independently of those in other groups. Vaccines that target these amino acids could, in theory, cut off the virus’s evolutionary escape route. In 2011, working with DNA sequence data from many strains of the virus, Chakraborty and Walker used random matrix theory (developed in the 1950s to study high-energy physics) to identify several such groups, including a particularly vulnerable group in the envelope surrounding the virus’s genetic material. They also found that T cells in patients who fight off HIV on their own disproportionately target the amino acids identified in the study. HIV strains with multiple mutations in these amino acids are rare, suggesting that those strains struggle to survive.

Building on that study, Chakraborty’s team is using a computational model that predicts how well viruses containing different protein variations can survive and reproduce. In the sequence of amino acids that makes up a protein, there are 20 ways to fill each “slot.” They analyzed thousands of HIV strains to calculate how much amino acid variation occurs in each of the approximately 500 slots in the proteins they studied—and how often any given sequence is seen. The more prevalent a sequence is, they showed, the more it must contribute to the virus’s fitness. With that data, they generated “fitness landscapes” in which protein sequences that strengthen the virus appear as hills, and those that weaken it are valleys. This knowledge can help guide the development of short protein sequences that, if delivered as vaccines, could make the virus less fit.
This may help scientists design vaccines not only for HIV but also for other viruses that mutate frequently. “If this holds up, then it’s exciting, because the two technologies you need to get fitness landscapes of viruses are sequencing and computation,” Chakraborty says. “Both of these technologies are going down in price very rapidly.”
Monitoring single cells
The ability to fight off HIV without drugs is rare, appearing in about one in every 300 people infected. By learning more about these “elite controllers,” Ragon Institute researchers hope to produce vaccines that mimic their success. To do that, scientists need a way to measure how individual immune cells respond to HIV-infected cells—something that was impossible until a few years ago.
Another MIT chemical engineer who attended the initial meeting with Walker and Ragon, Christopher Love, had recently developed a way to study how immune cells react to vaccines, food allergens, or infectious agents. He and Walker realized that this technology could be just what was needed to compare immune responses from HIV-infected people who resisted AIDS and those who became sick.
With Love’s system, single immune cells are placed in thousands of microscopic wells on a soft rubber surface. Secretions from each cell diffuse onto a slide placed on top, which is then tested for the presence of specific proteins such as inflammation-generating cytokines. Any proteins detected can be traced back to the cell by its “address” on the slide. This process generates a huge amount of data. “You can now make measurements on 10,000 cells and generate 20 to 30 parameters of data on each cell,” Love says.
In a 2011 study, Love and his colleagues analyzed T cells from HIV-infected patients to determine whether, as previous research had suggested, high levels of a cytokine called interferon gamma might correlate with the ability to kill HIV-infected cells. On the contrary, they found, T cells that secrete interferon gamma appear to kill HIV-infected cells only rarely.
Love now hopes to find biomarkers that reveal which T cells do target HIV effectively. He’s also studying antibody responses to HIV in immune cells from mucosal tissues lining the colon and reproductive tract. About half of human immune cells are in mucosal tissues, where most HIV infections occur, but most immunology studies are done with blood cells, so little is known about how mucosal cells function.
Love’s cell analysis system could let researchers track patients’ progress during clinical vaccine trials. To that end, he and colleagues are trying to boost its capacity from two daily sample sets (each of which contains 1,000 to 100,000 cells) to hundreds or thousands of sets. They are also working on software to help analyze the gigabytes of data drawn from each sample.
Walker is glad to have engineers working on health problems. “There are ways that we as clinicians think about scientific problems that can be limiting,” he says. “We think about what tools we have and what questions we can ask with those tools, whereas engineers say, ‘What question do you want to ask? Let’s build the tool.’”
Making vaccines more powerful
In the past 30 years, researchers have put three HIV vaccine regimens through large-scale clinical trials. Two proved ineffective, but one protected a small yet significant minority of subjects. “It does provide hope that a vaccine is possible,” says Ragon Institute member Darrell Irvine, a professor of biological engineering and materials science and engineering.
Most vaccines against diseases such as chicken pox and influenza use weakened forms of the virus as antigens, or substances that provoke an immune response. Because that’s seen as too dangerous for HIV, some researchers are making vaccines from one of its proteins. Others are injecting genetically engineered DNA into cells, where it can direct the production of viral proteins. DNA vaccines were first tested about 20 years ago and found to elicit strong immune responses in rodents. If successful in humans, they could be safer than conventional vaccines and could be stored longer. These vaccines would also be easier to develop for new diseases and to manufacture on a large scale, because researchers would simply program the DNA to produce the desired protein.
Injecting DNA or proteins on their own doesn’t always produce a strong enough immune response, so Irvine is studying two other strategies: adding an adjuvant, a molecule that helps stimulate the immune system, and delivering the antigen directly to the target immune cells.
Earlier this year, Irvine and colleagues developed a way to embed DNA in a polymer film. Microneedles deliver the film about half a millimeter under the skin—deep enough to reach immune cells that process infectious invaders in the epidermis, but not deep enough to hit nerve endings. Layers of the film gradually degrade, releasing the vaccine over days or weeks. The film also carries an adjuvant that provokes inflammation in the target tissue, attracting immune cells so they can encounter the DNA-encoded viral protein.
In studies of mice, the technique showed a promising ability to elicit an immune response. And in tests on skin samples from monkeys, the researchers found that DNA carried by the films got into skin cells much more easily—and appeared to persist longer—than DNA injected on its own. They are now hoping to conduct trials in nonhuman primates.
“[HIV is] a difficult problem, but I think it’s a solvable problem, and I think we’re obligated to bring [to it] every tool at our disposal,” Walker says. “The issues are how many resources do we have to put into this, and how quickly can we get it to the finish line as a scientific community?”
An Intellectual Hub for Medical Engineering
MIT’s new institute unites scientists, engineers, clinicians
In 1970, MIT and Harvard launched the Harvard-MIT Division of Health Sciences and Technology (HST), bringing together physicians, scientists, and engineers to transform the way medicine is taught and practiced. Now MIT has expanded on that mission by folding HST into a new Institute for Medical Engineering and Science (IMES).
Arup Chakraborty, the chemical-engineering professor who directs IMES, says it’s intended to serve as an “intellectual hub” that will better integrate HST with the rest of MIT and bring together disparate efforts in areas that contribute to medicine. It will pioneer new graduate-level curricula, offering certificates and possibly degree programs.
IMES now has 13 core faculty members—12 from HST plus Chakraborty. It will hire eight more, and all will divide their time equally between IMES and another MIT department to build connections across campus. IMES is also the home of MIT’s Medical Electronic Device Realization Center, recently launched to bring together MIT faculty, doctors, and industry representatives to develop better, less expensive medical devices.
In addition, IMES plans to work with Boston-area hospitals in strategic partnerships that may be modeled on the Ragon Institute and the Bridge Program, which links MIT researchers at the Koch Institute with physicians at the Dana-Farber Cancer Institute.
“If strategic partnerships evolve with area hospitals, then it will be building off of this proof of concept that the Ragon Institute has provided,” Chakraborty says. —AT
Keep Reading
Most Popular
Large language models can do jaw-dropping things. But nobody knows exactly why.
And that's a problem. Figuring it out is one of the biggest scientific puzzles of our time and a crucial step towards controlling more powerful future models.
How scientists traced a mysterious covid case back to six toilets
When wastewater surveillance turns into a hunt for a single infected individual, the ethics get tricky.
The problem with plug-in hybrids? Their drivers.
Plug-in hybrids are often sold as a transition to EVs, but new data from Europe shows we’re still underestimating the emissions they produce.
Stay connected
Get the latest updates from
MIT Technology Review
Discover special offers, top stories, upcoming events, and more.