Light Repels Light
Demonstrating a fundamentally new optical phenomenon, researchers at Yale University have shown the second half of an optical force that could make silicon photonics devices–such as those used in high-speed communications, network cards, even video and TV cables–faster and more capable.
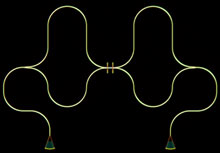
Results like these showing novel ways to control light “don’t come along very often,” says Oskar Painter, a microphotonics researcher at Caltech who was not involved in the work. “There’s a push to do more with optical components,” Painter adds, and the Yale group’s results are “totally new.”
Scientists theorized in 2005 that tiny beams of light confined on a silicon chip could attract or repel each other when placed in close proximity, similar to the electromagnetic forces between positive and negative charges. Last year a group led by Yale University professor Hong Tang first demonstrated the “attractive” side of this optical force. Now the group has demonstrated the second side of the force, repulsion, which makes its effects reversible.
Previously, says Mo Li, the lead author of the paper published in Nature Photonics, they could “pull” with the force, but they couldn’t “push.” Now the researchers can do both. The accomplishment opens the possibility of using light to manipulate light in microphotonic devices, rather than using mechanical elements like microheaters or power-hungry optical crystals.
Though the force is too weak to use on larger scales–two laser pointers couldn’t attract or repel each other, for example–the optical force operates strongly on the microscale, making it ideal for ultrahigh-speed, all-optical control of nanomechanical devices, according to MIT applied-mathematics professor Steven Johnson. In particular, Johnson points to the importance of being able to switch between attractive and repulsive optical forces, something that has not been experimentally demonstrated before.
Harnessing the optical force should enable faster data transfer in applications like fiber-optic telecommunications, where information can be encoded on multiple wavelengths of light and sped through a single fiber-optic cable in a process called wavelength division multiplexing. This process currently requires converting optical signals to electrical signals for modulation or amplification, and then converting them back to optical signals and sending them on their way. Using light to manipulate the optical signal could eliminate the need for electrical rest stops along the fiber-optic highway. “If you can directly transfer light to light,” says Li, “it will be cheaper and faster.”
Another problem with current optical multiplexing is that the devices that make the process work are relatively large–taking up prime real estate on silicon wafers–and they have to be engineered with strategically placed microheaters, which use changes in temperature to tune each wavelength of light just right. Such devices are slow and can cause cross-talk. Other light-manipulation techniques use special crystal materials that respond to high-intensity light to change the material properties of photonic devices.
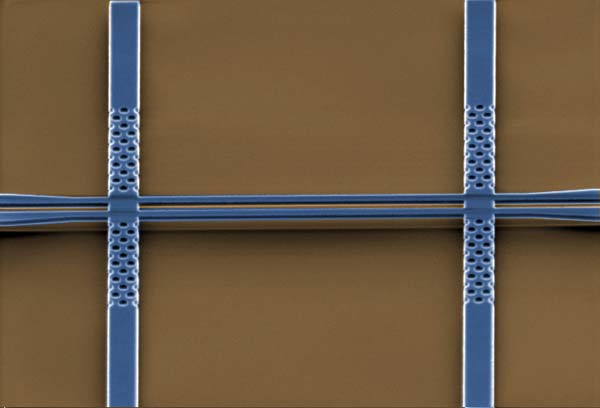
The Yale group’s approach demonstrates the possibility of manipulating one beam of light with another, right on the chip, without the need for slow, bulky heaters or external crystals. And because of their ability to harness both positive and negative forces, they can now effectively double the range of control over photonics circuitry.
The group used two identical waveguides–the optical equivalents of electronic wires, encasing the light beams moving through them–and suspended them in a central coupling region to allow them to move freely under the influence of the optical force. Then the researchers sent in a beam of laser light, split it in half, and forced one half through a longer path than the other. When the two halves of light recombined, they were out of phase because of having traveled different path lengths. The researchers found that when the light beams were out of phase, their waveguides repelled each other, but when the light was in phase, the waveguides pulled closer together. Because they could change the phase difference between the beams just by tweaking the wavelength of the input laser light, the researchers ended up with a new “knob” to control the optical force in a very simple step.
Though they weren’t transferring information or even turning switches on and off, the group successfully demonstrated the existence of–and easy flipping between–both sides of the force. Their next steps, says Tang, will be to build more complex circuits and improve the efficiency of their technique. They’ll also try to make the force stronger. “The bigger the force, the better,” Tang says.
The benefit of the Yale work, according to Caltech’s Painter, is that the researchers demonstrated the forces used for switching, but they also did it in a silicon system. That shows promise for future integration with microelectronics structures that are already processed on silicon chips. With the flexibility to control the forces right on the chip, key functionality would be added to the silicon microphotonics toolkit. The ultimate goal would be all-optical switches and devices, such as an optical bus that transfers information through a CPU with no electronic parts at all.
Keep Reading
Most Popular
Large language models can do jaw-dropping things. But nobody knows exactly why.
And that's a problem. Figuring it out is one of the biggest scientific puzzles of our time and a crucial step towards controlling more powerful future models.
How scientists traced a mysterious covid case back to six toilets
When wastewater surveillance turns into a hunt for a single infected individual, the ethics get tricky.
The problem with plug-in hybrids? Their drivers.
Plug-in hybrids are often sold as a transition to EVs, but new data from Europe shows we’re still underestimating the emissions they produce.
Stay connected
Get the latest updates from
MIT Technology Review
Discover special offers, top stories, upcoming events, and more.