First 3-D Map of a Fruit Fly’s Brain Network
An important goal in neuroscience is to understand the structure of links between neurons that make up the brain: in other words, to build an accurate 3-D map of the brain’s neural network.
Researchers have made significant progress with various kinds of high-resolution imaging techniques. For example, attaching fluorescent molecules to neurons can show their structure, and electron microscope images of brain sections can also reveal structure at the neuronal level.
These techniques all have an important limitation. The images they produce show changes in image intensity. But these variations must then be interpreted to infer the position and shape of the actual neurons. This final step is a difficult task when the neurons and the connections between them number in the thousands and millions.
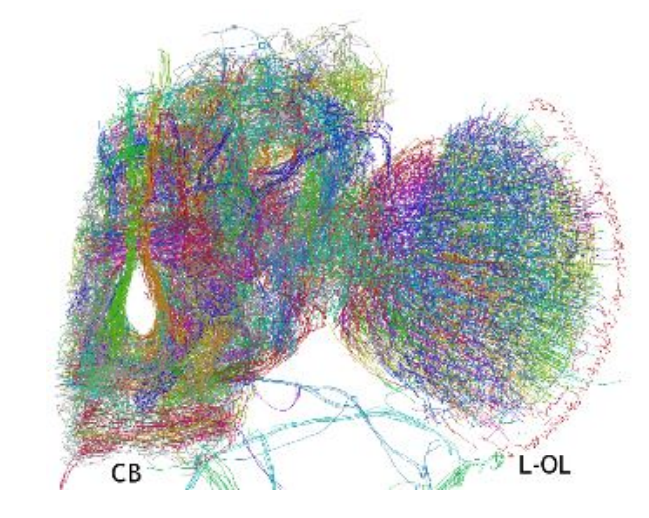
What’s needed is a better way of creating a 3-D wiring diagram of the brain—a kind of skeleton of the neural connections.
Today, that becomes possible thanks to the work of Ryuta Mizutani and pals at Tokai University in Japan. These guys have repurposed a technique for producing skeleton-like models of molecules and used it to map the neurons in a fruit fly’s brain. The result is the first 3-D model of this network of neurons.
The background to all this lies in biochemistry. Biochemists face a similar problem when they create 3-D models of complex molecules. They begin by creating a crystal of the molecule of interest. Then they zap it with x-rays and measure the diffraction pattern this forms, a technique known as x-ray crystallography.
But there is a problem. The x-rays are diffracted by the cloud of electrons that buzz around the atoms in the molecule. So the data reflects changes in electron density inside the molecule. The actual positions of the atoms have to be inferred from this data. When the structure is complex, this is not a simple task.
Chemists have a great deal of experience with this. For some years, they have used a computer modeling approach to solve the problem. It works by estimating the position of one atom in three-dimensional space, placing a node at that location in the model, and then connecting it by a virtual wire to the estimated position of the next atom, and so on. In this way, the software builds up a virtual wire model of the molecule.
Now Mizutani has repurposed this software to determine the 3-D position and shape of neurons. This is trickier because the neurons aren’t point-like objects like atoms but line-like objects that can twist and curve in complex ways.
The team gathers its data using a technique called x-ray tomography. They pickle a fly brain in a silver dye, bombard it with x-rays, and then measure the way the x-rays are scattered in various directions. This produces a 3-D image intensity map of the way silver in the neurons absorb x-rays.
The next step is the key: using the data to estimate the position and shape of the actual neurons. Mizutani and co place the data in a three-dimensional space of 840 by 1,250 by 1,200 voxels. They use the x-ray absorption intensities to estimate whether a neuron is present in particular voxel. Then they build a wire model by estimating how the neuron extends into any of the adjacent voxels.
Of course, the model has to check that the resulting network is consistent—that two adjacent neurons aren’t interpreted as a single extended neuron, for example. So the software continually checks the nature of the resulting network, looking for potential anomalies. Any anomaly that it can’t resolve is left for a human operator to fix.
This model has a resolution of around 600 nanometers and shows around 100,000 neurons, which the model resolves into about 15,000 traces. It was a significant effort for the team. “It took 1,700 person-hours to build the skeletonized model,” they say.
But the results are clearly worth it. The technique produces the first 3-D wire model of a fly brain hemisphere in which the position and shape of each neuron is mapped using 3-D Cartesian coordinates.
This model shows a wide range of known neuronal structures—the model mapped out 360 separate neuronal processes. But it also revealed a number of unknown structures that are clearly important. “These results suggest that neurons that cannot be classified into structural groups should play important roles in brain functions, though their structures have hardly been investigated,“ say Mizutani and co.
That points to some interesting work ahead, perhaps with shorter-wavelength x-rays that produce higher-resolution data.
However, the extra data will not be easy to handle, given that the current batch required so much work. “The reconstruction of [a higher-resolution] brain network appears to be prohibitively expensive in terms of human workload,” say Mizutani and co.
The data analysis is a significant bottleneck, and better automated model-building techniques are desperately needed. A clear opportunity for computer scientists with a bit of time on their hands.
Ref: arxiv.org/abs/1609.02261 : Three-Dimensional Network of Drosophila Brain Hemisphere
Keep Reading
Most Popular
Large language models can do jaw-dropping things. But nobody knows exactly why.
And that's a problem. Figuring it out is one of the biggest scientific puzzles of our time and a crucial step towards controlling more powerful future models.
How scientists traced a mysterious covid case back to six toilets
When wastewater surveillance turns into a hunt for a single infected individual, the ethics get tricky.
The problem with plug-in hybrids? Their drivers.
Plug-in hybrids are often sold as a transition to EVs, but new data from Europe shows we’re still underestimating the emissions they produce.
Stay connected
Get the latest updates from
MIT Technology Review
Discover special offers, top stories, upcoming events, and more.