Quantum Light Harvesting Hints at Entirely New Form of Computing
Physicists have long known that plants and bacteria convert light into chemical energy in a way that is hugely efficient. But only in recent years have they discovered that the molecular machines behind this process rely on quantum mechanics to do the job.
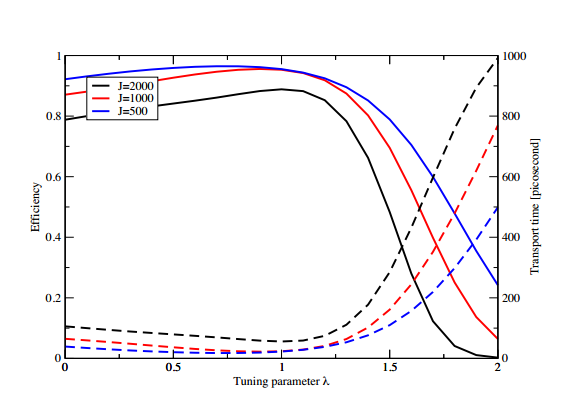
That’s a big surprise because of the temperatures involved. Quantum states are highly fragile—sneeze and they disappear in a puff of smoke. Physicists can maintain these states for some time in carefully controlled environments at low temperature, but nobody can explain how it can be possible in the warm wet environments inside living things.
Today, Gabor Vattay at Eotvos University in Budapest and Stuart Kauffman at the University of Vermont in Burlington have the answer. They say the processes behind light harvesting are a special blend of the quantum and the classical. And that this delicate mix represents an entirely new form of computing that nature might exploit in other systems too.
The quantum processes must studied in light harvesting systems occur in a structure known as the Fenna-Matthews-Olson or FMO complex, a huge pigment protein that is part of the light-gathering machinery in green sulfur bacteria. Embedded in these protein structures are reaction centres that convert the energy from light into chemical energy.
When light hits the FMO complex, the energy must travel across the protein matrix until it reaches a reaction centre. And amazingly, this transfer occurs with an efficiency of almost 100 percent.
That’s puzzling because the only way for the light energy to find a reaction centre is to bounce through the protein network at random, like a ricocheting billiard ball. This process would take too long, much longer than the nanosecond or so it takes for the light energy to dissipate into the environment and be lost.
So the energy transfer process cannot occur classically in this way. Instead, physicists have gathered a variety of evidence showing that the energy transfer is a quantum process.
The thinking goes like this. Because energy can exist in a superposition of states, it can travel a variety of routes around the network at the same time. And when it finds the correct destination, the superposition collapses, leaving the energy at the reaction centre. The result is an almost perfect transfer of energy.
But Vattay and Kauffman say that this kind of pure quantum process this cannot be responsible either. That’s because a number of quantum processes slow down the movement of quantum objects through random networks like this. “Quantum mechanics has adverse effects too,” they say.
One of these party-poopers is known as Anderson localisation, a phenomenon that prevents the spread of quantum states in random media. Because the quantum state acts like a wave, it is vulnerable to interference effects, which prevent it propagating in a random network.
Another is the quantum zeno effect, the paradoxical phenomenon in which an unstable state never changes if it is watched continuously. That’s because watching involves a serious of measurements that constantly nudge the state, preventing it from collapsing. This is the quantum version of the watched-pot-never-boils effect.
A similar thing happens to the quantum state of the energy during light harvesting. This quantum state will inevitably interact with the environment but these interactions act like measurements. This triggers a quantum zeno-like effect that prevents the state from collapsing at the reaction centre. So the energy transfer cannot occur in this way, say Vattay and Kauffman.
Instead, they propose a new process in which the quantum search mechanism and the interaction with the environment combine to overcome Anderson localisation. It is the interplay between these processes that delivers the energy to the reaction centre in an optimal way, they say.
The idea is that the interaction with the environment changes the wave-like nature of the quantum state just enough to prevent Anderson localisation. At the same time, the quantum zeno effect extends the life time of the quantum state allowing it to find its way to the reaction centre. It is this interplay between the quantum and classical worlds that allows the energy transfer.
That explains the quantum-like behaviour of light harvesting processes at room temperature. But Vattay and Kauffman say the idea has other important implications. The problem of finding a reaction centre in a protein matrix is formally equivalent to many other problems in computing. So it ought to be possible to turn light harvesting to the task of computing by mapping one problem onto the other.
That could dramatically improve computational speeds at room temperature. “Computers based on artificial light harvesting complexes could have units with 100-1000 times larger efficiency at room temperature,” say Vattay and Kauffman.
What’s more, this kind of computation may already be at work in nature. “Since the realization of this mechanism seems now relatively easy, it is an important question if it has been realized in light harvesting systems or is also present in other biological transport or optimization processes. Especially in the human brain,” they say.
If they are right, this new kind of computation could generate a flurry of interest in a short space of time.
Ref: arxiv.org/abs/1311.4688: Evolutionary Design in Biological Quantum Computing
Keep Reading
Most Popular
Large language models can do jaw-dropping things. But nobody knows exactly why.
And that's a problem. Figuring it out is one of the biggest scientific puzzles of our time and a crucial step towards controlling more powerful future models.
How scientists traced a mysterious covid case back to six toilets
When wastewater surveillance turns into a hunt for a single infected individual, the ethics get tricky.
The problem with plug-in hybrids? Their drivers.
Plug-in hybrids are often sold as a transition to EVs, but new data from Europe shows we’re still underestimating the emissions they produce.
Stay connected
Get the latest updates from
MIT Technology Review
Discover special offers, top stories, upcoming events, and more.